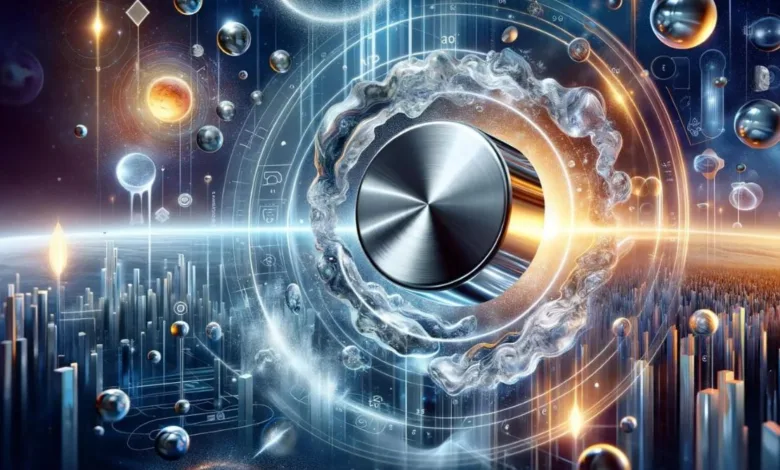
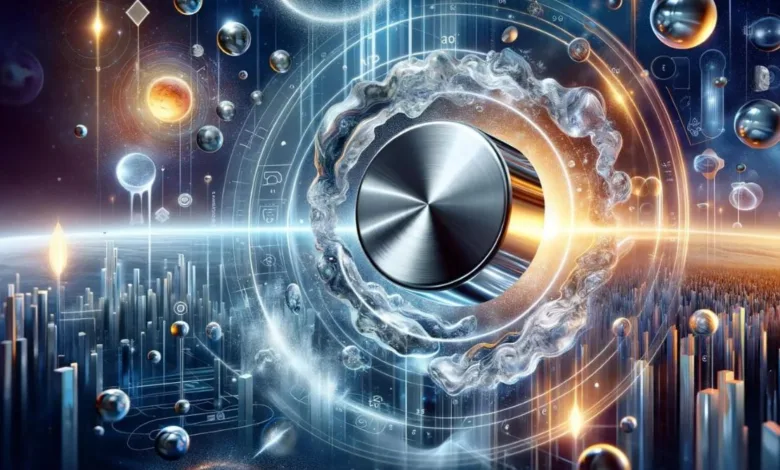
Beyond the Core: Sodium’s Astonishing Transformation and the Quest for Deeper Scientific Understanding
Sodium’s Astonishing Transformation|: Delving deep below the Earth’s surface or into the heart of the sun triggers remarkable transformations in matter at the atomic level. stars, planets’ intense pressure can turn metals into non-conducting insulators, as demonstrated by the intriguing metamorphosis of sodium from a gleaming, gray metal to a glass-like insulator transparent when subjected to sufficient pressure.
A recent investigation led by the University at Buffalo has unveiled the chemical bonding intricacies behind this extraordinary high-pressure phenomenon. Contrary to the previous notion that high pressure pushes sodium’s electrons in a space between atoms. Quantum chemical calculations by the researchers reveal that these electrons remain firmly connected to the surrounding atoms through chemical bonds.
“We’re responding to an extremely straightforward inquiry of why sodium turns into a separator, yet foreseeing how different components and synthetic mixtures act at exceptionally high tensions will possibly give knowledge into greater picture questions,” makes sense of Dr. Eva Zurek, a teacher of science at the UB School of Expressions and Sciences and co-creator of the review
The study not only confirms but also expands upon the hypothetical forecasts of the late physicist Neil Ashcroft, to whom the research is dedicated. Two decades ago, Ashcroft and Jeffrey Neaton challenged the conventional belief that materials always adopt a metallic state under high pressure, presenting evidence that certain materials, including sodium, can become separators or semiconductors when compressed.
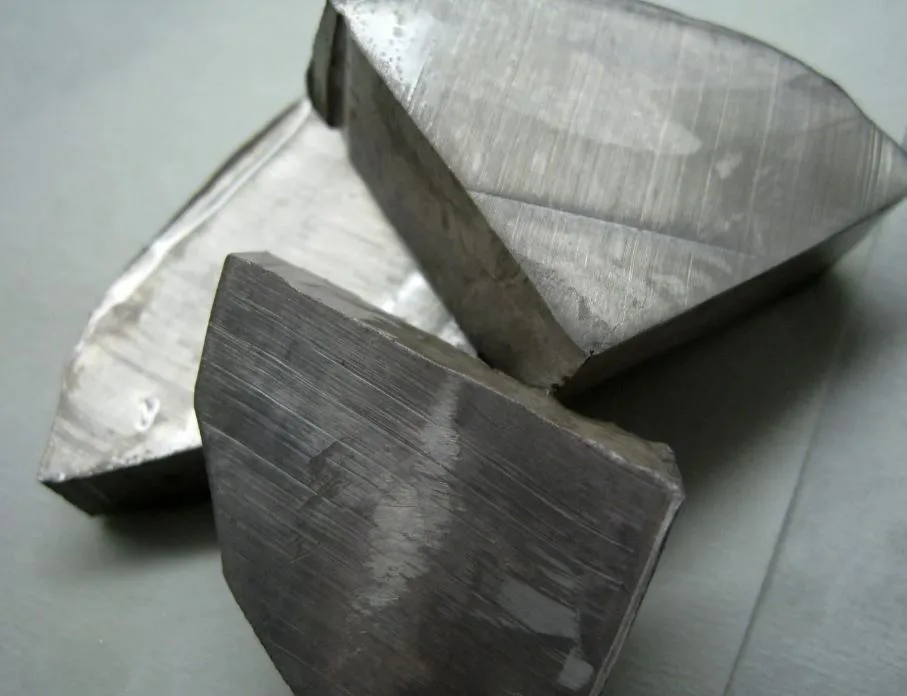
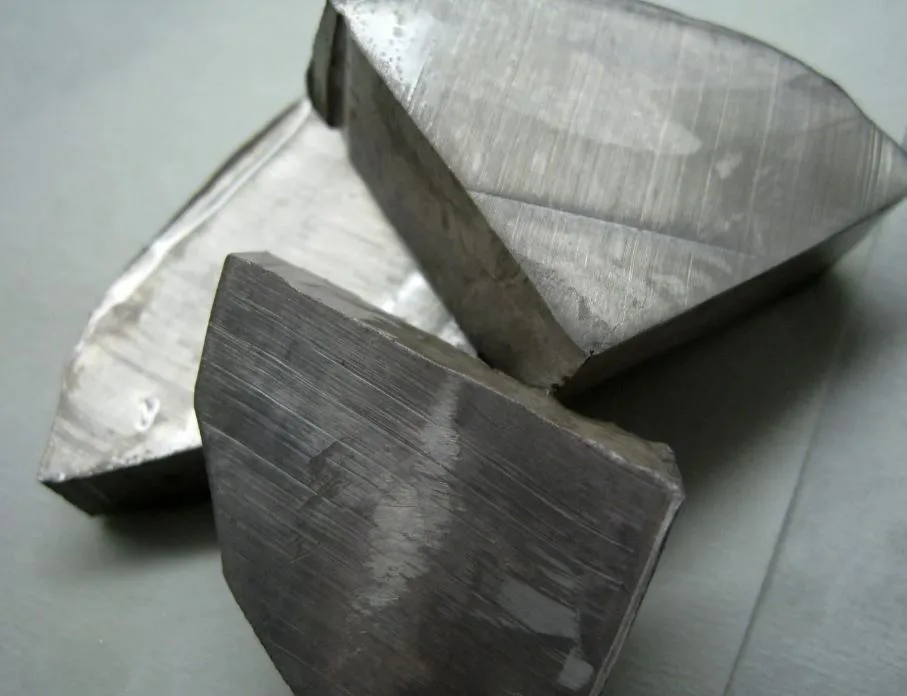
Lead author of the UB-led study, Dr. Stefano Racioppi, emphasizes that their work goes beyond the physics perspective presented by Ashcroft and Neaton, associating it with substance bonding concepts. The research employed supercomputers at UB’s Center for Computational Research to simulate the behavior of electrons in sodium atoms under high pressure, as replicating such pressures in a lab setting below Earth’s crust can be challenging.
The simulations revealed the emergence of an electride state, where electrons are trapped in the interspatial regions between atoms. This phenomenon explains sodium’s transition from a reflective metal to a translucent cover, as free-streaming electrons retain and retransmit light, while caught electrons permit light to go through.
Crucially, the calculations demonstrated interestingly that the arrangement of the electride state can be explained through substance holding. High tension prompts electrons to involve new orbitals inside their separate particles, and these orbitals overlap to form chemical bonds, resulting in localized charge concentrations in the interstitial spaces.
As opposed to before presumptions that high strain powers electrons out of particles, the new results revealed that the electrons remain part of the encompassing iotas. “We understood that these are not simply disengaged electrons that chose to leave the particles. All things considered, the electrons are divided among the particles in a synthetic bond. They’re quite special,” notes Racioppi.
Contributors to the study incorporate Malcolm McMahon and Christian Tempest from the College of Edinburgh’s School of Physical Science and Cosmology and Community for Science at Outrageous Circumstances.
The research received support from the Place for Issue at Nuclear Strain, a Public Science Establishment focus drove by the College of Rochester, focusing on how pressure within planets and stars can alter the atomic structure of materials.
Dr. Zurek acknowledges the challenges of replicating conditions deep within Jupiter’s atmospheric layers but underscores the use of calculations at times, high-level lasers to recreate these circumstances.
This groundbreaking research not only answers the fundamental question of sodium’s transition to an insulator but also paves the way for a deeper understanding of the behaviors of various elements and compounds under extreme pressures, shedding light on broader inquiries about the interior of stars, the generation of planets’ magnetic fields, and the evolution of stars and planets.